By Katie McCulloch, New Science Communicator Guest Blogger
615+ Million years ago.
Parrsboro, Nova Scotia (ish).
Earth.
The sun shines down on an unfamiliar landscape. The Earth looks different, from its barren surface to the arrangement of the continents. What will eventually become part of Nova Scotia is unrecognisable and volcanically active. Of particular interest, to this storyteller, at least, is a relatively small mass of molten rock, slowly rising from deep within the Earth’s crust. Over millions of years, it inches its way upwards, slowly cooling as it travels.
Fast-forward to a scorching August day, summer 2015. We are in the Cobequid Highlands just north of Parrsboro, Nova Scotia. The trees give precious little shade as I and another researcher trek up the stream bed, weighed down with hammers, notebooks, and backpacks full of rocks, eyes fixed on the bedrock at our feet. It’s the last day for collecting rocks and observations for my thesis, and I’m grateful for my fellow student’s help. Along with our heavy loads, we have an absurd number of horseflies to contend with, as well as numerous bogs, downed trees, and waterfalls to circumvent.
Ah, science.
A pluton forms when rocks that have melted deep in the Earth’s crust migrate upwards, but cool and solidify without reaching the surface.
We have reached the final resting place of the molten blob. After millions of years, the overburden has been eroded, exposing the now cold and very solid body of rock at the surface. This mass of bedrock, with surface dimensions of just 1 × 3 km, is called a pluton, and is completely different from the rock surrounding it. A pluton forms when rocks that have melted deep in the Earth’s crust migrate upwards, but cool and solidify without reaching the surface. Their melting is initiated by some event – a reduction in pressure, increase in temperature, or the introduction of water. The small amount of rock that melts slowly worms its way upward. The events that cause melting at depth cause deformation above, providing an area for the magma to accumulate. This can happen many times, until an enormous mass of magma has been emplaced and cooled.
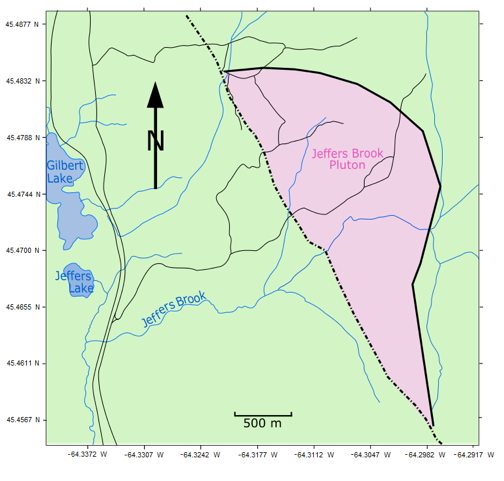
This is a simplified map showing the shape and extent of the pluton at the surface. The solid line indicates an intrusive contact— the dotted line is a probable fault. Image: Katie McCulloch, used with permission.
The pluton is far from its origins deep in the Earth, both in distance and time. What clues can it give us about its past, and the geological history of this place?
The answer is quite a few. The major challenge here is the same as with any undertaking in geology – almost everything we want to know is either hidden underground, or was once above our heads and has since been eroded away. Erosion erases the rock record, leaving enormous gaps. Geologists piece together the history of the earth from the miniscule percentage of rocks that are available for us to sample and examine. We do this by learning from experiments, comparing what we see to modern analogues, and making educated guesses. We then attempt to paint a picture of the past that contains more blank space than brushstrokes.
What clues does our subject give us to work with, and how do we fill in the blanks?
If we take a walk across the pluton, we can see it is made up of a patchwork of similar rocks, varying slightly in colour and texture. Like leaves from the same tree in autumn, no two are perfectly identical, but the similarities are easy to see.
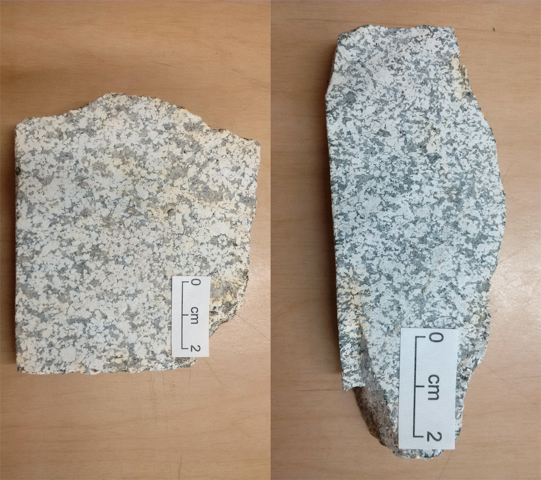
These rock samples have been sawed into a 2 cm thick slabs, for ease of examination. The similarities between rocks are evident. Photos: Katie McCulloch, used with permission.
Within this broader patchwork, various dark and light rock fragments, from the size of a finger to larger than a whole hand, can be spotted throughout the pluton. As the pluton slowly pushed its way upward, it forced its way into cracks in the surrounding rocks, which then broke off and became suspended in the rising magma. So, despite never having drilled in this area, we can deduce that matching rocks must exist somewhere in the vicinity.
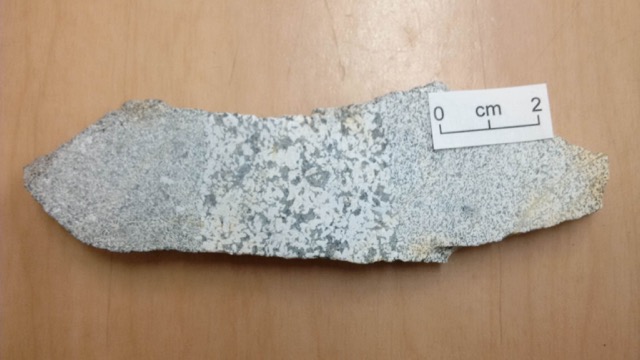
This rock slab was trimmed to show two different rock fragments (left, right) taken in by the molten rock as it rose. They’re called xenoliths. Photo: photos to Katie McCulloch, used with permission.
Under a microscope, we see that the rock is composed of interconnected crystals. Many minerals have the same basic composition but different crystal structures, and some crystal structures only form under a specific amount of pressure. This means that certain minerals will only form when pressures are great enough, the general rule being that pressure increases with depth. This fact can be used to help determine the depth at which the pluton cooled.
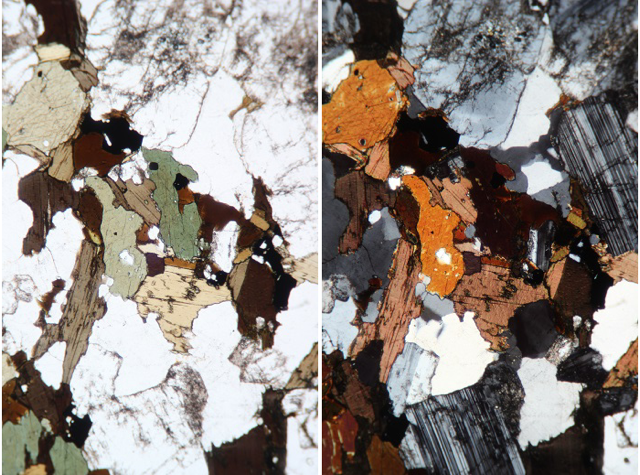
The left photograph, taken through a microscope, is of a slice of rock 0.03 mm thick. The transparent minerals are quartz and plagioclase; the greens and browns are amphibole and biotite. The photograph on the right was taken with a polarizing filter applied. This helps significantly with mineral identification. Photos: Katie McCulloch, used with permission.
To delve even deeper into the rock’s makeup, we rely on lab equipment that tells us which elements are present. We crush fist-sized samples into a fine rock flour, and send them away to be analyzed. The data we get in return are incredibly useful – by plotting the ratio of one element to another, or the total amount of an element in a rock, we can get an idea of what sort of geological environment the pluton formed in. In this case, evidence confirms that, millions of years ago, this area probably looked a lot like volcanically active Japan.
Finding out whether or not there is a relationship between these plutons will contribute to our understanding of the geological history of Nova Scotia.
Knowing the chemical composition of each rock sample also makes it easy to compare rocks beyond visual similarities. For example, a recent study analyzed numerous samples of Neoproterozoic-era (1000 to 541 million years old) plutons west of where I worked. Comparing the chemical compositions of those plutons to that of the one I am studying will indicate if there are any relationships between them, a sort of geological DNA test. If the plutons came from the same place or event, no matter the distance between them, we can find that relationship. That is what I’m currently trying to do.
Finding out whether or not there is a relationship between these plutons will contribute to our understanding of the geological history of Nova Scotia, and from there, we can better understand how it fits into the bigger picture. The more we can learn about the origins and relationships between rocks, the better we can unravel and understand our planet’s complex geological history.